For applications in spin-based electronics and quantum computation, it is crucial to understand quantitively how the electron spin is coupled to the orbital degrees of freedom. In bilayer graphene this is a notoriously difficult task, given the tiny size of the energy scales involved. Researchers from RWTH Aachen University have now managed to accurately measure the spin-orbit coupling in single-electron bilayer graphene quantum dots, exploiting the extreme energy sensitivity of a double-dot device. The result has been reported in Nature Communications.
The device used for this experiment is a particularly sophisticated one (see Fig. 1). A sheet of bilayer graphene is encapsulated within two crystals of hexagonal Boron Nitride (hBN), and placed on a graphite back-gate. On top three layers of metallic gates allow to form two tiny potential wells – the so-called quantum dots – and to force electrons to pass through them one by one.
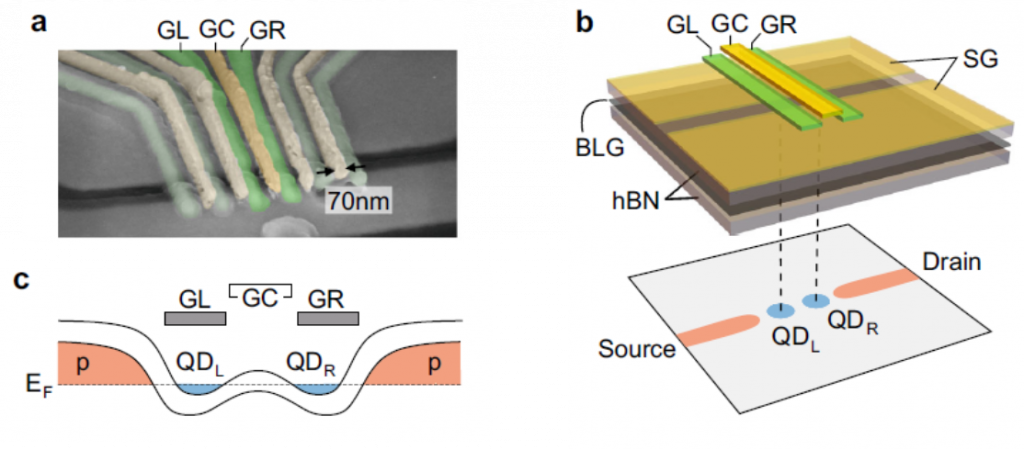
By tuning the device in a regime where at most one electron is allowed into each dot and by measuring the current as a function of magnetic field (see Fig. 2), the RWTH team has been able to reconstruct the single particle spectrum of both quantum dots with great accuracy.
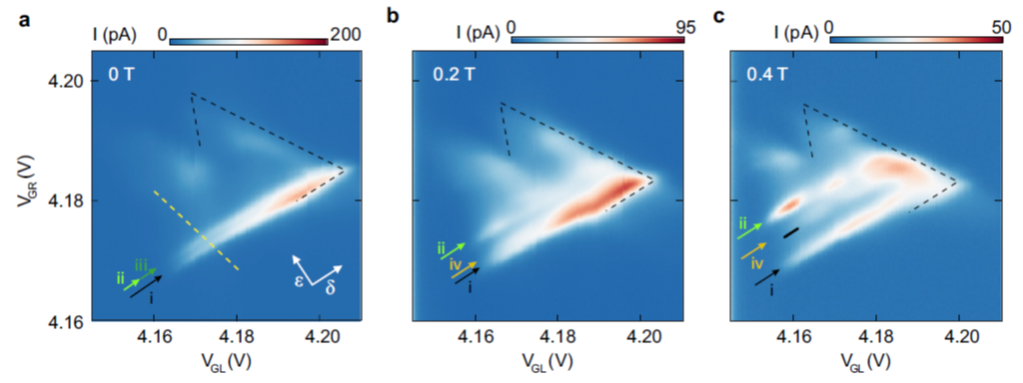
Figure 2 Current through the device as a function of gate voltage, in the regime in which each dot can be occupied at most by one electron. The lines of enhanced current correspond to configurations in which electronic states in the two dots are energetically aligned. By measuring the separation between the different lines as a function of the applied magnetic field, it is possible to reconstruct the single-particle spectrum of the dots. The spin-orbit coupling leads to the splitting of the ground-state at zero magnetic field (see black and green arrows in panel a).
In particular, Banszerus and co-authors could accurately estimate the strength of the spin-orbit coupling in the system and show that it is of Kane-Mele type. This type of spin-orbit coupling is intrinsic to graphene and bilayer graphene, and it is particularly interesting because it gives rise to a topologically protected ground state. “We extract a value of about 60 meV for the spin-orbit coupling in bilayer graphene”, says Banszerus. “This is more than two times higher than what predicted by theory for pure bilayer graphene. We believe the discrepancy comes from the fact that in our devices the bilayer graphene is actually encapsulated between hBN, and that the enhancement is caused by proximity effects.”
The same type of experiments also put an upper bound of 20 meV to a possible disorder-induced mixing of the two-valleys of the graphene spectrum. “This small value is not entirely unexpected”, explains Banszerus, “as in this type of the devices the graphene sheet is extremely flat and free of disorder. But it is a good news, because without valley-coupling the existing spin-orbit coupling might be used for future qubit operations.”
Bibliographic info:
Spin-valley coupling in single-electron bilayer graphene quantum dots
L. Banszerus, S. Möller, C. Steiner, E. Icking, S. Trellenkamp, F. Lentz, K. Watanabe, T. Taniguchi, C. Volk, and C. Stampfer, Nature Communications 12, 5250 (2021).